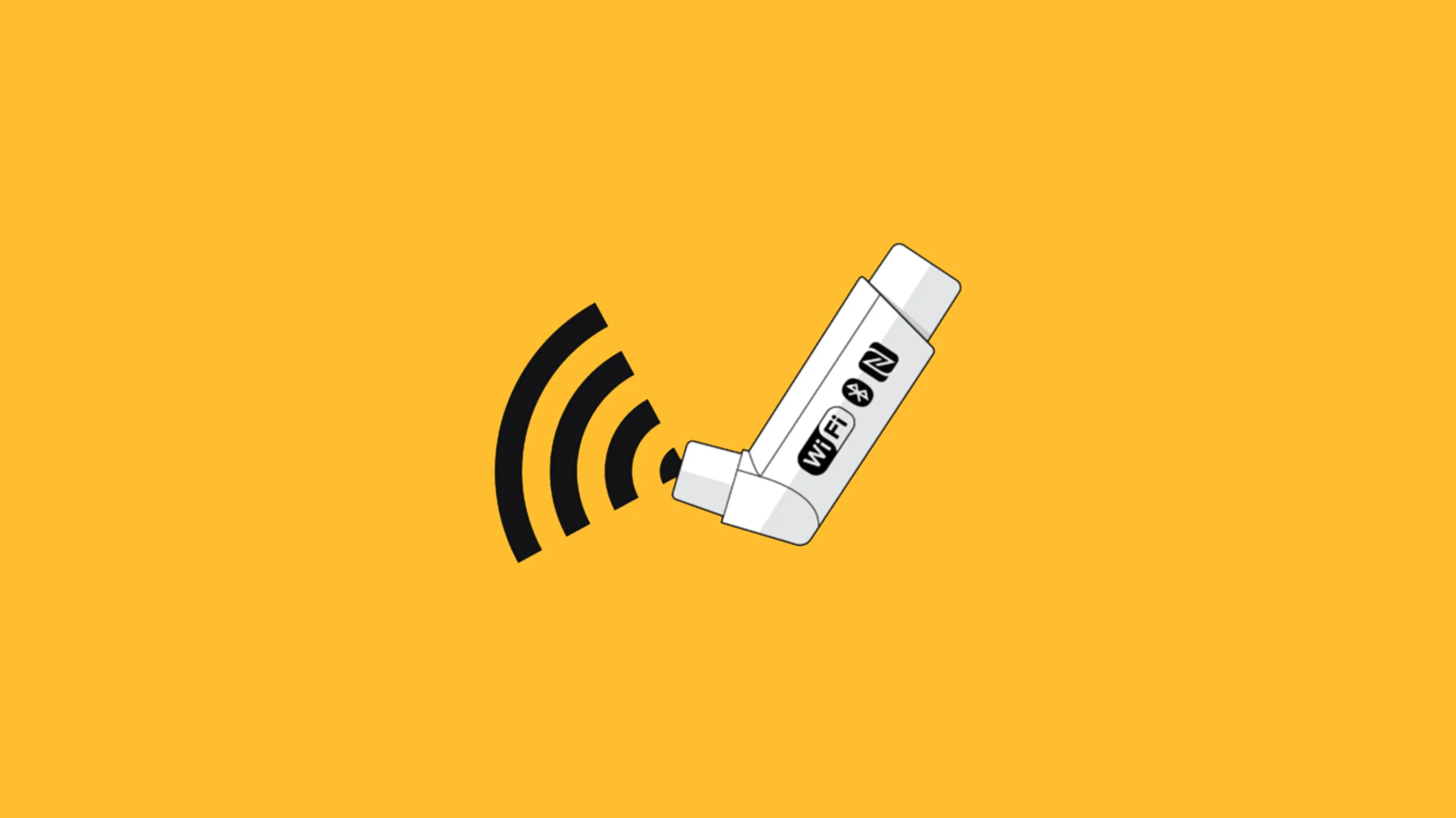
Don’t develop a connected drug delivery device without reading this
Here, Matt Jones, Senior Sector Manager, Medical and Scientific, DCA – along with colleagues Richard Gledhill, Aidan O’Hare, Tony Smith, James May, Daniel Jenkins and Rob Veasey – summarises some of the key challenges and opportunities in the development of connected drug delivery devices, focusing in detail on deciding what a connected drug delivery device should do, designing for a coin cell, direct cellular connection and antenna design.
The market for connected drug delivery devices is in its infancy but many predict that it will grow at an astonishing rate (ref 1). At DCA, we think this is likely to happen – but only if these devices can demonstrably improve the lives and outcomes of the patients who use them.
The advent of new and exciting technology has brought with it a temptation to connect anything to everything, providing features that sometimes offer little real benefit. In this context, we believe the value proposition for new connected drug delivery devices must be clearly established at the outset.
One school of thought is that new connected objects should be created and launched to see what emergent behaviours develop and which products win in the market. Some manufacturers opt to develop a ‘minimum viable product’ by simply connecting an existing product and exploring how users respond to the new proposition. This gets products to market fast – increasing the likelihood of taking early market share and even of establishing a new connected ‘ecosystem’.
This ‘fail fast’ approach has many success stories, particularly in the consumer products market, where development time, development costs, product lifespan and inherent product risk are often low.
For drug delivery devices, the picture is different. To make it to market, new products need to be demonstrably safe and effective. Regulators demand extensive evidence of this and fears around data security and privacy are often far more critical than with consumer products. This creates challenges that are in turn compounded by the rapid evolution of underlying connectivity technologies.
So what’s the alternative to this ‘suck it and see’ approach? As obvious as it may sound, we think the best answer is found by first explicitly considering and defining the need before developing the product.
Why connect?
The ability to pass information to or from a drug delivery device enables features that can train and guide patients, monitor their usage, record side effects or assist in managing regimen changes. All of this can allow interventions to improve patient compliance and reduce risks. It is also likely to change business models by providing data that facilitates outcome-driven payments.
What should a connected drug delivery device do?
It can be tempting to collect and present data simply because it is accessible. However, in the context of a medical device, it is imperative that the data is relevant, useful and accessible to everyone who will interact with it – whether they be a patient, carer, doctor or payer.
The functionality of connected devices should be informed by a detailed understanding of the conditions that they need to support. One place to start is by considering the use of the non-connected legacy version of the product. Observing and interviewing stakeholders can reveal rich insights.
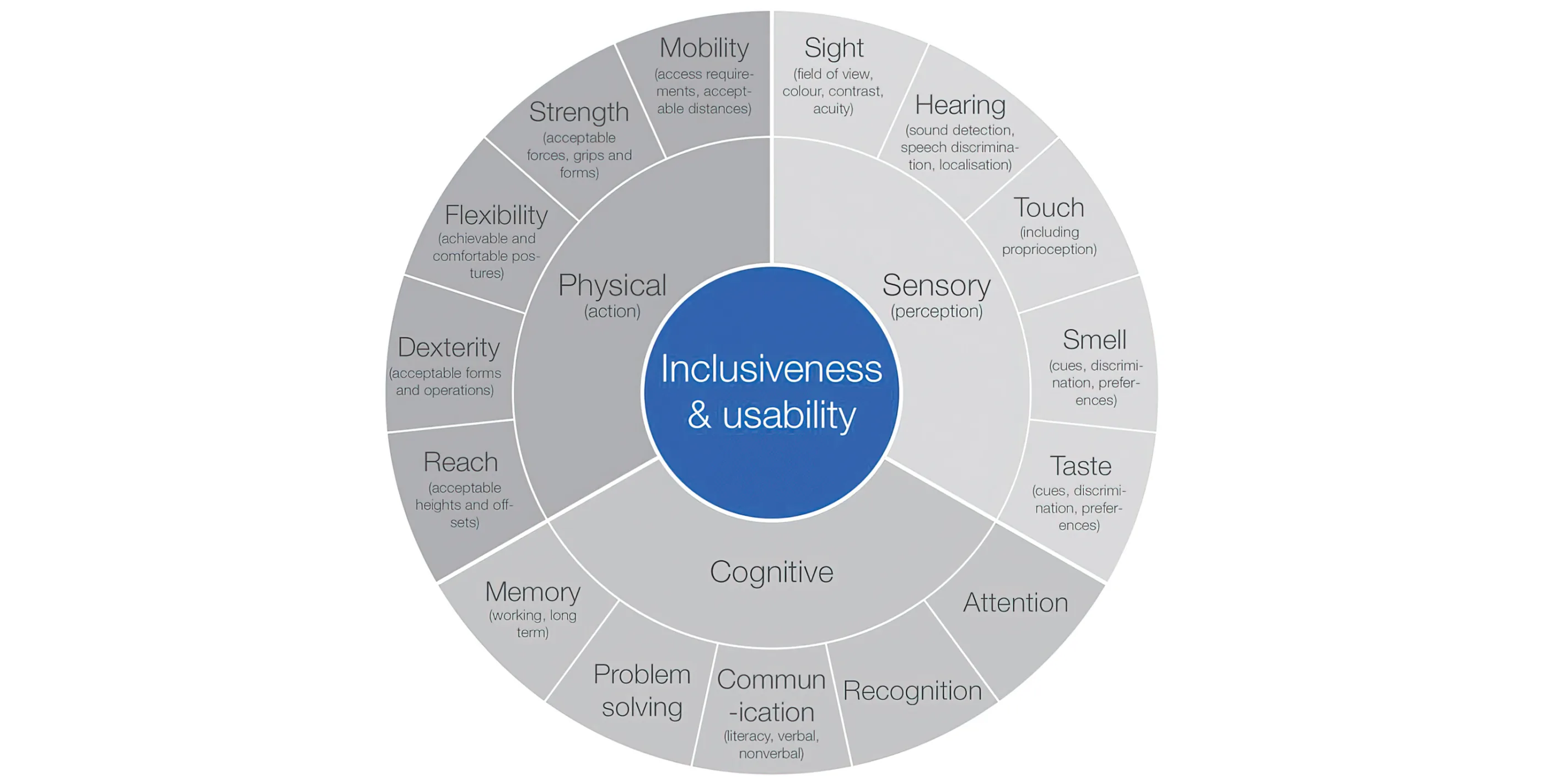
Figure 1 - Breaking down inclusiveness and usability
The international standard for medical device usability (ISO 62366-1) advocates the use of task analysis to evaluate activities. This involves breaking down the activity into sub-tasks, which are then themselves broken down until base-level operations are reached. Each base-level task can be examined to assess the demands it places on the user at a sensory, cognitive and physical level see Figure 1.
This usability analysis will often throw up where new features or information can help support the user in carrying out a particular task. One approach is to then map the functionality of the system in a tabular form, comparing the legacy system with the proposed new connected system as shown in Figure 2.
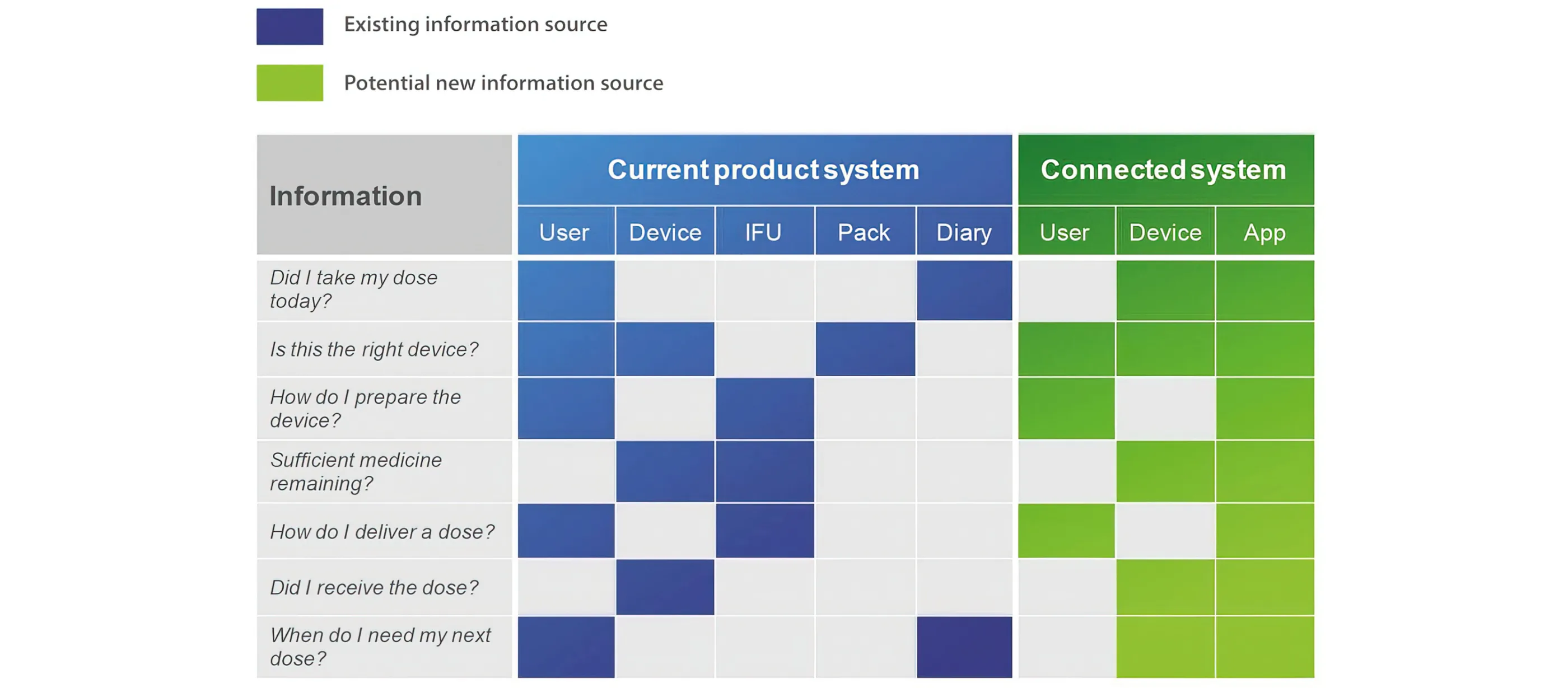
Figure 2 - Comparing an existing system to its connected equivalent
The alternative or additional features or information sources can then be explored, assessed and filtered based on the benefits and potential risks to the stakeholders.
Generating a reasoned, useful and safe set of features is more likely to yield a successful connected drug delivery device if the development team follows a structured process aligned to the relevant standards (e.g. ISO 13485, EN 62304, EN 62366 and ISO 14971). This should support an understanding of the needs and limitations of all stakeholders and help establish the device feature set at the start of the design process. This can then be reviewed and iterated through development.
The next step is to translate this design input into a successful device concept. For a connected drug delivery device, the development process often revolves around a few key challenges, first among which is usually the power source. There are many options available to the developer of a connected drug delivery device – off-the-shelf batteries, bespoke and flexible batteries, energy harvesting, printed batteries, etc. But for reasons of size, cost and availability, a simple, non-rechargeable coin cell is often selected.
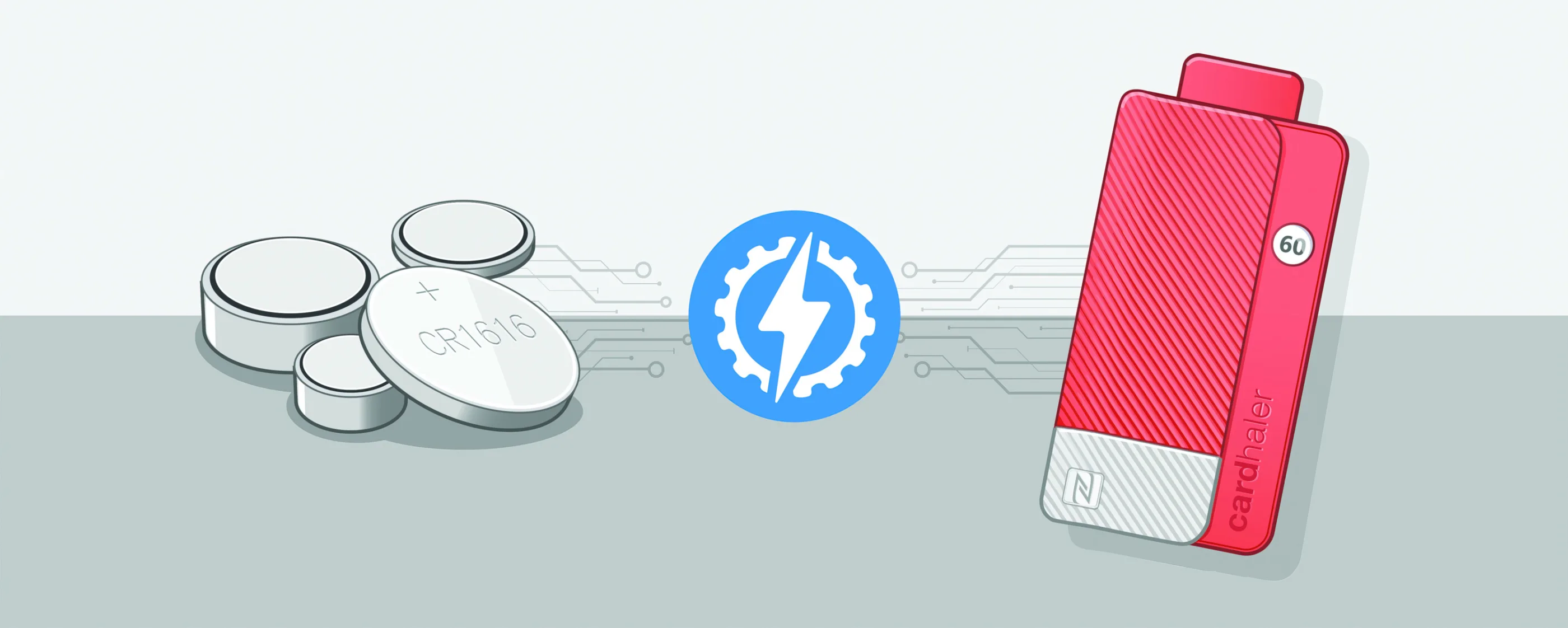
Figure 3 - Designing for a coin cell
Designing for a coin cell
For all its benefits, the very limited current capability and capacity of coin cells pose challenges that affect every aspect of the device design.
Sensors and Storage
In one example, measuring physical characteristics such as pressure changes in a connected inhaler might be accomplished by using an optical transmitter and receiver. However, running an optical transmitter such as an LED continuously takes more current than the battery can provide. Pulsing the transmitter with short bursts of energy is an option. However, this can only go so far; if the pulses become too short, the transmitter no longer acts like a simple on/off switch and analogue side effects begin to make readings unreliable. At this point, a more sophisticated approach is required, such as pre-charging a capacitor to improve the turn-on speed of the LED and make the best use of the pulse length available.
Once this problem has been solved, it is possible to take readings. Storing this information to non-volatile data storage would, in normal circumstances, be considered a relatively trivial exercise. However, when the memory is full, erasing data can use up considerable power, depending on the specification of the storage component. One potential solution is to dimension the storage memory to prevent the need to erase any records.
Data Transmission
Once the measurements have been stored, this data often needs to be sent to a paired mobile device. The tiny current capability of a coin cell can generally be managed by the use of suitable capacitors between the battery and the RF transmitter chip. However, the leakage current through these capacitors can drain the battery. Therefore, the amount of data transmitted must be minimised to limit the number of capacitors and consequently the cost, printed circuit board (PCB) space required and background current drain.
Similarly, care needs to be taken when transmitting (relatively) large amounts of data. Sending a single reading may only be a small number of bytes but synchronising a device with a user’s new phone could easily result in a few thousand records being requested by the app. Sending that much data too fast could drag the battery voltage down, so intelligent throttling of the flow of data may be required.
The User Interface
With the widespread adoption of mobile phones and their large displays, developers often decide to use a more basic interface on the device itself, such as a flashing LED or a sounder. This seems simple, yet the coin cell’s low voltage may mean it’s not capable of directly driving the LED.
It may be necessary to pulse the LED using a charge-pump system, for example, and vary the pulse duty cycle and speed depending on the battery voltage to achieve a consistent brightness.
Start-up
To achieve the battery life required for a disposable device, the microprocessor must require an incredibly low current while the device is not in use. This means in most cases that the device is effectively coming out of reset each time, rather than a standby mode, and has to go from a standing start to taking the first measurements very quickly. This can be challenging and may drive the selection of an appropriate microprocessor.
Battery Life – Beginning and End
At the beginning of its life, microprocessor programming during manufacture can use up too much of the coin cell’s valuable energy unless carefully handled. As the battery comes towards the end of its life, its nominal (unloaded) voltage may appear reasonable but as soon as any current is drawn from it, it may drop significantly, potentially below the minimum operating voltage of the microprocessor. This could affect references and ranges for analogue inputs, the performance of sensors, and Bluetooth transmissions. Switched-mode power supplies will have to work harder to generate stable voltage supplies, further increasing the load on the battery and exacerbating the problem. If these issues are not handled gracefully, various parts of the system may spontaneously reset or misbehave.
Finally, there is the issue of managing power ‘on the shelf’ between manufacture and first use. One option is to have a small strip of pull-tape between the battery and its contacts but this risks moisture ingress. It might also be necessary to have some changing data retained from manufacture, such as a real-time clock value. In either case, the current drawn must typically be virtually zero before first use to achieve an acceptable in-use life.
Designing for a Coin Cell: Summary
All aspects of the design of a connected drug delivery device that uses a coin cell are likely to revolve around the coin cell itself. This is fundamentally different from designing devices with larger battery packs or a permanent power supply.
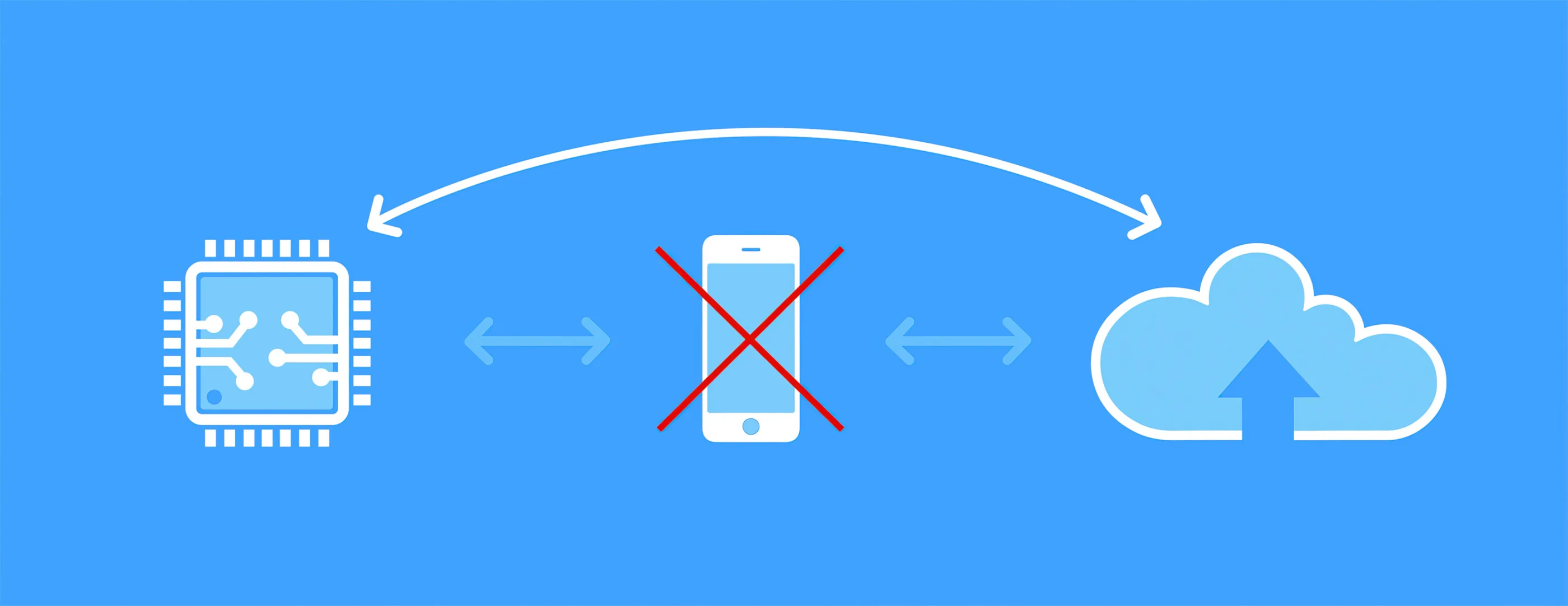
Figure 4 - New low power IoT chipsets offer exciting opportunities
New communication protocols bring exciting opportunities
A major part of the power budget of a connected drug delivery device is determined by the wireless communication protocol. Bluetooth Low Energy (BLE) and near field communication (NFC) are current favourites but new communication protocols are continually emerging.
Currently, to connect our intelligent devices to the internet, we are dependent on the presence of a local network on our phones and tablets or around our buildings. However, exciting developments are being made in the shape of new low-power cellular Internet of Things (IoT) chipsets that use one of two new radio transmission methodologies: 4G LTE-M and NB-IoT. They replace the current short-range wireless capability (e.g. BLE or Wi-Fi) with a direct cellular connection. This means devices no longer need to be connected through a phone or added to a local network (Figure 4) – opening up the potential for a wide range of new device features, vastly improved usability and greater adoption.
The worldwide network infrastructure to support 4G LTE-M and NB-IoT is currently incomplete but coverage is rapidly increasing. These new communication protocols provide opportunities to redefine the next generation of connected products and user experiences by:
- Eliminating dependency on phones, Wi-Fi routers, etc. to form the intermediate communications link
- Enabling lower power location of devices
- Removing the need for multiple platform apps (e.g. for Apple or Android)
- Simplifying the process of establishing a connection, which can be problematic for some users.
- Providing greater opportunities for tighter data security control at the device
- Improving the host server’s connection to the device, offering new feature opportunities (e.g. alerts if emergency medications are used).
The hardware platforms to support this functionality are now being made commercially available. This opens up opportunities to use their functionality to provide new features and benefits to the end user.
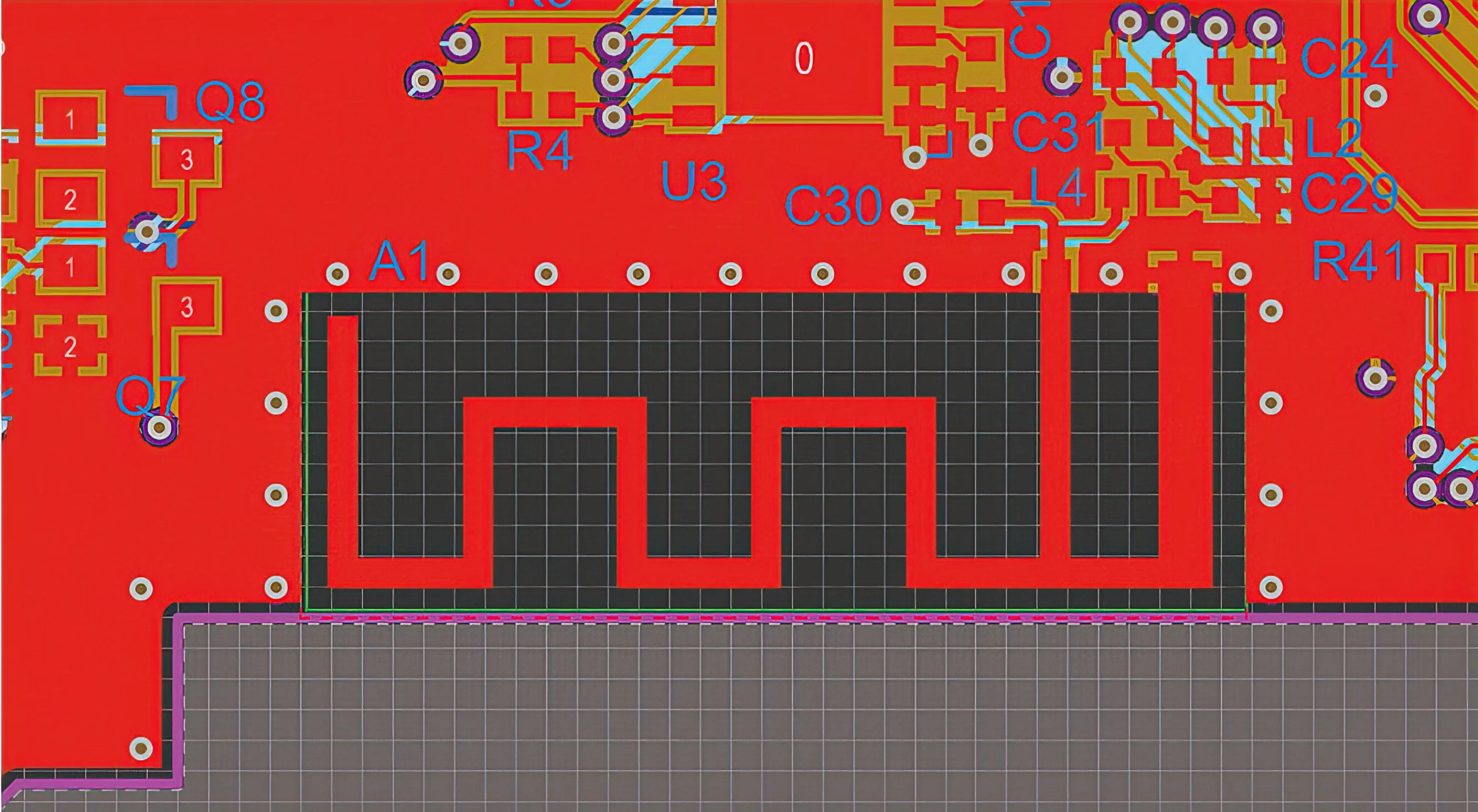
Figure 5 - Antenna design is critical for connected devices
Antenna design
The antenna is a key part of the product architecture for connected devices – while it is part of the electronic circuit, it also has a large influence on product packaging and mechanical design. As such, it is essential that the electronic, mechanical and visual design are all addressed simultaneously.
Large metal components near the antenna – such as batteries, motors or PCB ground planes – will reduce wireless communications range. If the product is handheld, poor antenna placement can lead to problems if the antenna can be masked by the user’s hands or even touched. Even if an off-the-shelf RF module with an integrated antenna is used, poor location of this within the device can severely reduce range.
Where device cost is particularly sensitive, it is attractive to use a printed antenna on the PCB. However, the board area required for the antenna itself and the necessary separation from other parts of the circuit can be significant and cause the PCB to grow, affecting the overall product size or form factor. A surface mount component can be used with a smaller footprint than a printed antenna but this introduces additional parts and still limits the placement options to locations within the PCB footprint. By contrast, designing a bespoke antenna that is soldered to the PCB but extends beyond the board footprint can improve its location.
There may be an existing metallic part in the device that can serve a dual purpose as the RF antenna – or, conversely, an external finish that degrades performance. The most appropriate approach needs to be evaluated on a case-by-case basis, as effective product design is about striking a balance between all functional characteristics of the device.
Conclusion
Connected drug delivery devices offer a huge range of opportunities but come with an equal or greater number of challenges, which must be carefully negotiated to bring them to market successfully. Maximising the probability of success depends on deploying an experienced team and following a structured, evidence-based process. Due to highly constrained design challenges, multidisciplinary development teams must be tightly integrated and focused on common and well-understood goals. In this way, companies can deliver connected devices that really have the potential to improve people’s lives.
Reference
- “Connected Drug Delivery Devices Market Worth $717.7 Million By 2025”. Grand View Research, 2018.
This article was originally published in the June 2019 issue of ONdrugDELIVERY magazine.